Unveiling the Power of GPON in Modern FTTH Networks
FTTH (Fiber to the Home) is a fiber optic access technology that directly connects residential or corporate users, enabling a direct link from service providers to the end user. Meanwhile, GPON (Gigabit-capable Passive Optical Network), which provides data transmission via fiber optics, is usually the preferred choice in FTTH networks. This post mainly discusses the advantages of GPON in the FTTH access network and the components and architecture of GPON networks.
What is GPON?
GPON is a type of fiber-optic broadband network technology that allows for the delivery of high-speed internet, voice, and video services to homes and businesses. In GPON networks, data signals are transmitted over long distances using optical fibers, providing users with faster and more reliable connectivity compared to traditional copper-based networks. GPON operates on a passive optical network architecture, which means it utilizes passive optical components like splitters and combiners to distribute signals without the need for powered equipment along the transmission path.
Why Is GPON a Preferred Choice in FTTH Network?
Enhancing Home Network Connectivity
GPON technology supports downstream speeds of 2.5 Gbps and upstream speeds of 1.25 Gbps. This capability allows household members to engage in various online activities such as HD video streaming, online gaming, and video conferencing simultaneously. Furthermore, it supports multiple devices and users within a household to be online concurrently without compromising network performance, ensuring each family member can enjoy their online experiences without interruption.
Coverage Range Fits FTTH Deployments
GPON technology supports long-distance transmission, typically reaching up to 20 kilometers from the central office to the subscriber’s premises. This extensive reach allows service providers to cover a wide geographic area with a single fiber optic network, minimizing the need for additional infrastructure investments. As a result, GPON is well-suited for various deployment scenarios, especially in Fiber to the x (FTTx) networks.
Cost-Effective Through Passive Architecture
GPON adopts a passive network architecture, which means the optical devices it employs do not require power supplies, thus reducing dependence on energy and active equipment. Meanwhile, the prices and failure rates of passive equipment are much lower than those of active devices, resulting in a corresponding reduction in overall operational costs. Furthermore, GPON networks utilize passive splitters to achieve a point-to-multipoint (P2MP) network architecture, significantly reducing deployment costs while ensuring signal quality. Therefore, GPON technology offers a highly cost-effective passive architecture in FTTH networks, allowing customers to maximize benefits with minimal investment costs.
Scalability and Reliability
The GPON network boasts high scalability, enabling service providers to effortlessly expand their networks to accommodate growing user bases. With the continuous evolution of PON networks, the optical network units (ONUs or ONTs) within users’ homes can seamlessly transition to support higher-speed equipment in the future, such as units compatible with 10G-GPON, XGS-PON, or NG-PON2 standards. This facilitates increased bandwidth without the need to modify existing fiber infrastructure. This is to say, despite evolving bandwidth demands and internet technologies, existing FTTH infrastructure can reliably adapt and upgrade, ensuring the continuous delivery of efficient network services.
Components of GPON FTTH Access Network
In a GPON FTTH access network, there are three main components: optical line terminal (OLT), optical splitters, and optical network terminal (ONT).
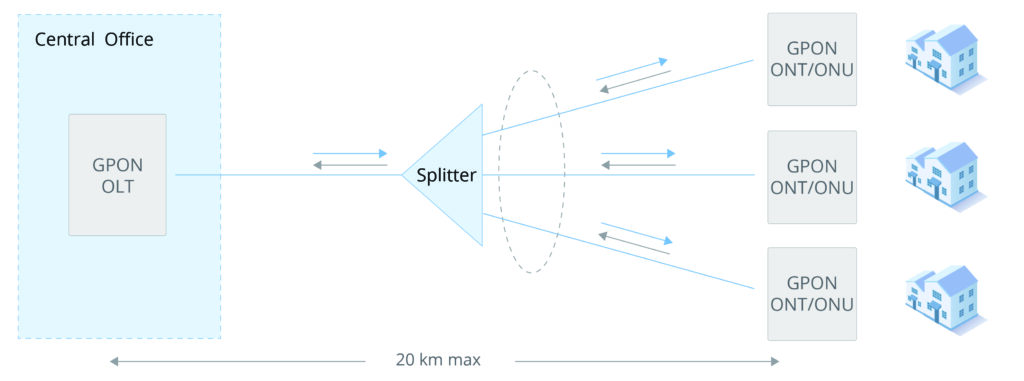
OLT (Optical Line Terminal)
The OLT functions as the endpoint for service providers in a passive optical network, typically situated in a data center or main equipment rooms Serving as the powerhouse for FTTH systems, the OLT transforms optical signals into electrical signals, presenting them to a core Ethernet switch. In doing so, it replaces multiple layer 2 switches at distribution points. The OLT’s distributed signal connects to backbone cabling or horizontal cabling through optical splitters, establishing links with optical network terminals at individual work area outlets.
ONT/ONU (Optical Network Terminal/Unit)
Deployed at customer premises, the ONT/ONU connects to the OLT via optical fiber, with no active elements in the link. In the GPON network, the transceiver within the ONT/ONU establishes the physical connection between customer premises and the central office OLT. The ONT/ONU serves as the interface for end-users, facilitating the conversion of optical signals back into electrical signals for seamless connectivity to various devices such as computers, phones, and televisions in a Ffiber-the-home(FTTH) network.
استفاده از امواج میلی متری 60 گیگاهرتز توسط مراکز ارائه دهنده اینترنت جهت افزایش سرعت اینترنت وایرلس به سرعتهای قابل رقابت با اینتزنت فیبر نوری
با پیشرفت تکنولوژی و تولید انواع رادیوهایی با امواج میلی متری شرکت های ارائه دهنده اینترنت نیز میتوانند با جایگزینی رادیوهای 60 گیگاهرتز سرعت اینترنت را برای هر یوزر به یک گیگابیت بر ثانیه، نرخ دیتا تجمیعی دو طرفه را به 3.5 گیگابیت بر ثانیه برسانند که این سرعت کاملا قابل مقایسه با سرعت اینترنت روی فیبر نوری است. این سرعت از آن جهت اهمیت دارد که شرکت های ارائه دهنده اینترنت عمدتا به فیبر دسترسی ندارند و برای رقابت با شرکت های مخابراتی که شبکه فیبر ایجاد کرده اند نیاز به سرعت های بیشتر جهت رقابت دارند. از آنجایی که آنتن های 60 گیگاهرتز با گین بالا بسیار کوچک هستند وقتی که با آنتن های 5 گیگاهرتز و2.4 گیگاهرتز مقایسه شوند فضای کمتری را روی دکل یا پشت بام یا در حالت تکیه به دیوارخواهند گرفت
: همچنین تجمع تعداد زیادی رادیو بر روی دکل به دلیل استفاده از آنتن های زیر امکان پذیر است
Narrow Beamwidth
Beamforming
به همین دلیل یک حسن دیگر رادیو های 60 گیگاهرتز توانایی اجتناب از تداخل خودی و تداخل با بقیه فرکانس هاست. از طرف دیگر به دلیل فرکانس بالا برد آنها از 150 متر تا 5.2 کیلومتر است که عدد اول برای مناطق شهری پر تراکم و عدد دوم برای مناطق خارج شهر بدون هیچ مانع می باشد که موجب میشود تعداد رادیوها در مناطق متراکم شهری بیشتر شود که این امر یک حسن هم دارد و آن استفاده مجدد از همان فرکانس است که به افزایش ظرفیت سرویس دهی شرکت های ارائه دهنده اینترنت می انجامد
ولی یکی از اشکالات فرکانس60 گیگاهرتز متأثر شدن شدید آن از بارندگی است که برای این وضعیت هم تدبیر اندیشیده شده است و آن استفاده از یک رادیوی 5 گیگاهرتز که به صورت توکار در همان محوطه قرار دارد و به محض شرایط اضطراری ( بارندگی ) به صورت اتوماتیک و بدون قطعی جریان تامین اینترنت به مشتریان را بدست میگیرد
New Technologies behind the Gigabit Wireless OFDMA, 1024 QAM, MU-MIMO, TWT and BSS Coloring
Introduction: From 802.11 to 802.11ax
The base version of the IEEE 802.11 standard debuted in 1997 with specifications for data rates of 1 and 2 megabits per second (Mbit/s). Since then, the Institute of Electrical and Electronics Engineers (IEEE) has ratified 5 major iterations of the 802.11 Wi-Fi protocol, culminating with 802.11ac in 2013. However, despite a significant increase in speed, many organizations are finding themselves limited by the 802.11ac standard, particularly in high-density venues such as stadiums, convention centers, transportation hubs and auditoriums. To meet the challenges of high-density deployments, the IEEE recently introduced the 802.11ax standard, which is backward compatible with previous generations of Wi-Fi, to deliver faster network performance and connect more devices simultaneously. With an expected four-fold capacity increase over its 802.11ac Wave 2 predecessor, 802.11ax is successfully transitioning Wi-Fi from a ‘best-effort’ endeavour to a deterministic wireless technology that is fast becoming the de-facto medium for internet connectivity. Indeed, 802.11ax access points (APs) deployed in dense device environments will support higher service-level agreements (SLAs) to more concurrently connected users and devices – with more diverse usage profiles. This is made possible by a Range of technologies that optimize spectral efficiency, increase throughput and reduce power consumption. These include Orthogonal Frequency-Division Multiple Access (OFDMA), Target Wake Time (TWT), BSS Coloring, 1024-QAM and MU-MIMO
OFDMA
Current and older iterations of Wi-Fi are client-centric with a randomized, contention-based approach that relegates Wi-Fi to a “free-to-send” first come, first served paradigm which is untenable for modern high-density deployments. In contrast, OFDMA is AP-centric and enables an 802.11ax access point to simultaneously communicate with multiple devices by dividing each Wi-Fi channel into smaller sub-channels known as Resource Units (RU). The access point determines how to allocate the sub-channels, as each individual RU (or sub-channel) can be utilized for different clients that are serviced simultaneously. In other words, an AP can choose to allocate the whole channel (all sub-channels within a channel) to a single user in a given time frame – or it may partition the whole channel to serve multiple devices simultaneously. This technique improves the usable throughput for all devices connected to an AP.
The allocation and scheduling capability supported by OFDMA allows multiple devices to receive more consistent attention. This reduces the use of the contention methodology that preceded 802.11ax and moves Wi-Fi from a contention-based to a scheduled-based service, which is analogous to an unmanaged four-way street intersection finally getting a traffic light. This helps stabilize Wi-Fi performance, especially in higher-density environments such as stadiums, convention centers, transportation hubs, and auditoriums.
1024 QAM
QAM (quadrature amplitude modulation) is pronounced as a one-syllable word that rhymes with Guam. QAM has to do with the way that digital information is transmitted between two points. Fundamentally, QAM enables an analog signal to efficiently transmit digital information. It also provides the means by which an operator transmits more bits in the same time period, which effectively increases the bandwidth.
QAM is a modulation scheme that transmits data by changing the amplitude, or power level, of two signals: first in-phase with the incoming data and the second 90 degrees out of phase. QAM relates to the number of bits of information encoded in each time period. For example, eight bits defines the number of combinations that are possible for those two signals (in phase and 90 degrees out of phase). If there are 256 combinations possible for those eight bits, then it is referred to as 256 QAM. Using a single time period to convey 10 bits would be 1024 QAM.
While the older Wi-Fi 5 (802.11ac) standard is limited to 256-QAM, the new Wi-Fi 6 (802.11ax) standard incorporates an extremely high optional modulation scheme (1024-QAM), with each symbol (a point on the constellation diagram) encoding a larger number of data bits when using a dense constellation. In real-world terms, 1024-QAM enables a 25% data rate increase (throughput) in Wi-Fi 6 (802.11ax) access points and devices. With over 30 billion connected “things” expected by 2020, higher wireless throughput facilitated by 1024-QAM is critical to ensuring quality-of-service (QoS) in high-density locations such as stadiums, convention centers, transportation hubs, and auditoriums.
MU-MIMO Multiuser-MIMO
UL MU-MIMO is a new key feature introduced with Wi-Fi 6 (802.11ax). By leveraging UL MU-MIMO, multiple clients connected to the access point will be able to send acknowledgment responses (ack) simultaneously, thus saving airtime. This ultimately improves network throughput and efficiency.
TWT – Target Wait Time
Target Wake Time enables devices to determine when and how frequently they will wake up to send or receive data. Essentially, this allows 802.11ax access points to effectively increase device sleep time and significantly conserve battery life, a feature that is particularly important for the IoT. In addition to saving power on the client device side, Target Wake Time enables wireless access points and devices to negotiate and define specific times to access the medium. This helps optimize spectral efficiency by reducing contention and overlap between users.
BSS Coloring
Legacy high-density Wi-Fi deployments typically saw multiple access points assigned to the same transmission channels due to a limited amount of spectrum – an inefficient paradigm that contributed to network congestion and slowdowns. Moreover, legacy IEEE 802.11 devices were unable to effectively communicate and negotiate with each other to maximize channel resources. In contrast, Wi-Fi 6 access points are designed to optimize the efficient reuse of spectrum in dense deployment scenarios using a range of techniques, including BSS Coloring. This mechanism intelligently ‘colour-codes’ – or marks – shared frequencies with a number that is included within the PHY header that is passed between the device and the network. In real-world terms, these color codes allow access points to decide if the simultaneous use of spectrum is permissible because the channel is only busy and unavailable to use when the same color is detected. This helps mitigate overlapping Basic Service Sets (OBSS). In turn, this enables a network to more effectively – and concurrently – transmit data to multiple devices in congested areas. This is achieved by identifying OBSS, negotiating medium contention and determining the most appropriate interference management techniques. Coloring also allows Wi-Fi 6 access points to precisely adjust Clear Channel Assessment (CCA) parameters, including energy (adaptive power) and signal detection (sensitivity thresholds) levels